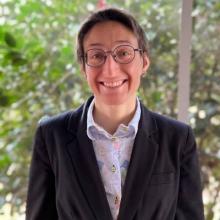
Dr. Chloé Arson is the Susan G. and Christopher D. Pappas Professor in the School of Civil and Environmental Engineering (CEE) and an adjunct faculty in the School of Earth and Atmospheric Sciences at the Georgia Institute of Technology (Georgia Tech). She earned her Ph.D. at Ecole Nationale des Ponts et Chaussées (France) in 2009. Arson’s primary expertise is in damage and healing rock mechanics and multi-scale modeling of coupled deformation and fracture processes in geomaterials. Her group’s scientific contributions span the fields of engineering mechanics, computational mechanics, geoscience, and bio-inspired design. Her research has been funded by federal and state agencies, industry, domestic and foreign research institutions, and Georgia Tech, totaling $8.2M in research grants. Arson published 76 articles in leading journals of computational geomechanics, applied mechanics and geoscience. She received the NSF CAREER (2016) and NSF BRITE (2021) awards. Website: http://arson.ce.gatech.edu/
Landscapes encode the history of the climate. For example, saprolite, the intermediate material between rock and soil, plays a critical role in the evolution of topography, nutrient supply, landslide hazards, and the global carbon cycle. The subsurface also bears resources used for the production of energy and construction materials. It is thus important to assess the response of soils, rocks and other geomaterials to a varying climate and to increasing societal demands. Here, we present analytical and numerical models to predict the propagation of microscopic cracks and metric fractures in porous media subjected to variable mechanical, hydraulic and chemical conditions. We first explain a micro-mechanical model of anisotropic damage induced by the weathering of biotite minerals. The bedrock is modeled as a matrix that contains and anisotropic distribution of microscopic cracks and biotite inclusions endowed with a weathering stress field, called eigenstress. The mechanical properties of the bulk rock are obtained by homogenization. With this model, we conduct a series of finite element simulations in bedrock under gently rolling topography and a range of biotite orientations. In our simulations, crack growth and stress redistribution are far more sensitive to biotite weathering than to the topographic or regional stresses, which suggests that biotite weathering is capable of dominating the development of bedrock damage. We deploy cohesive zone (CZ) elements at the boundary of the finite elements and show that biotite weathering can affect the trajectory of metric fractures. Furthermore, we propose a strategy to simulate multi-scale fracture propagation coupled with fluid flow with the extended finite element method (XFEM) and simulate a series of injection tests in an inclined fracture embedded in a transversely anisotropic porous medium. The fracture propagates along the bedding direction in the absence of in situ stress and along the direction of maximum compressive stress when anisotropic stress boundary conditions are applied. The proposed XFEM model allows
simulating multiscale hydraulic fracturing. To upscale the transport properties of the bedrock from the microscopic to the metric scale, we then extend the theory of homogenization to diffusion problems by using imperfect interfaces. We develop a self-consistent model for halite that explains why rock made up of larger crystals exhibit lower creep deformation, while specimens with smaller grains have a lower diffusivity. Simulations show that grain boundary healing accelerates specimen compaction, while precipitation in the pores controls the evolution of effective diffusivity. These results are impactful for geological storage design, but it remains challenging to formally link the microstructure changes induced by chemical reactions and pore deformation to macroscopic physical and mechanical properties, because the key microstructural features that govern macroscopic fluid flow differ from those that dominate elastic, plastic and brittle behaviors. We thus conclude this talk by discussing Artificial Intelligence strategies to extract microstructure features from various image data.