Computational Mechanics Projects
Principal Investigator(s):
H. Alicia Kim
Researcher(s):
Sandiliya Kambampati
Summary:
We developed a level set topology optimization method for 3D cooling channels, considering convective heat transfer for high Reynolds number flows. The Darcy potential flow is used to simulate the flow using the finite element method. The resulting velocity field is used in a convection-diffusion model to simulate the heat transfer using the finite element method. The pressure drop and the average temperature are considered as the objectives, which are minimized subject to a volume constraint and a maximum length scale constraint. Our results show that the pressure drop and the average temperature are conflicting criteria. We show an example in 3D where we optimize the cooling channel topology that conforms to the surface of a sphere.
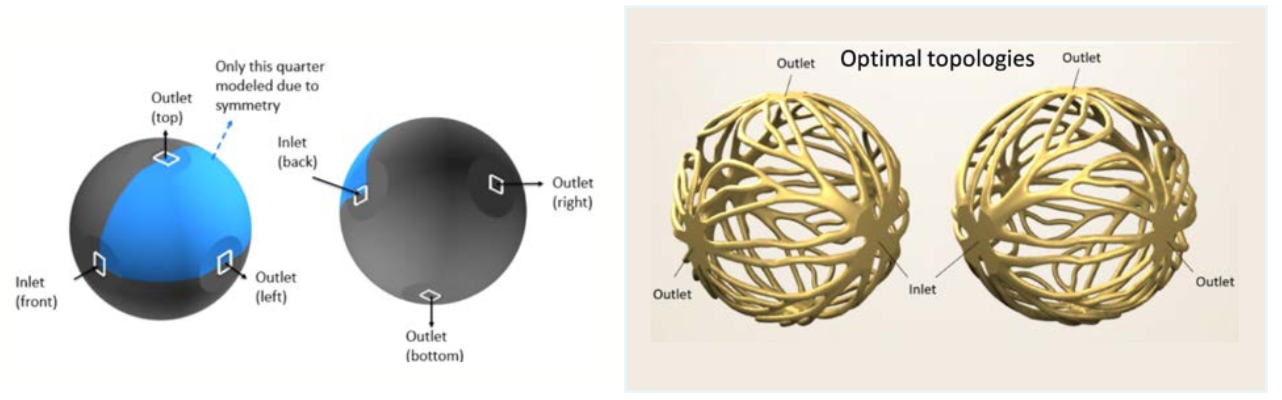
Principal Investigator(s):
Petr Krysl
Summary:
Methodology for stabilizing mean-strain hexahedron and tetrahedron finite elements for applications to anisotropic deformation in the infinitesimal- and finite-strain was described in several papers by Krysl et al. The approach is based on a sampling of the stabilization energy using the mean-strain quadrature and the “full” integration rule. This combination is shown to guarantee consistency and stability. The stabilization energy is expressed in terms of input parameters of the real material, and the value of the stabilization parameter is determined in a quasi-optimal manner by linking the stabilization to the bending behavior of the elements.
The accuracy and convergence characteristics of the stabilized mean-strain formulations for both solid and thin-walled structures (shells) compare favorably with the capabilities of mean-strain and other high-performance hexahedral and tetrahedral elements described in the open literature and also with a number of successful shell elements.
Principal Investigator(s):
J. S. Chen
Summary:
The complex multi-scale failure modes, damage evolution, and fragmentation resulting from high velocity contact-impact processes in solids and structures pose considerable difficulties in simulations using finite element methods. J. S. Chen is one of the original developers of meshfree Reproducing Kernel Particle Method (RKPM) for modeling material damage in fragment- impact processes. RKPM is formulated with state-of-the-art stabilization and variationally consistent methods, which ensures the stability, accuracy, and efficiency in the numerical simulation. The in-house Nonlinear Meshfree Analysis Program (NMAP) developed based on RKPM by Chen’s group has been successfully applied to the modeling of Empty Room Closure at the Waste Isolation Pilot Plant (sponsored by the Sandia National Laboratories) as shown in Fig. 1, where the shearband formation and damage accumulation associated with the closure of an underground disposal room (rock salt repository) under the natural compaction over hundreds of years are simulated. Funded by the National Research Council Canada, the RKPM capabilities have been applied to the simulation of reinforced concrete column under blast load as shown in Fig. 2, where RKPM simulation accurately captures the formation of diagonal cracks on the column’s shaft as well as the post-blast intense spalling as observed by the UCSD blast simulator. Sponsored by the National Science Foundation, Prof. JS Chen’s group has developed an efficient RKPM reduced-order modeling technique for the fast prediction of thermal fatigue behaviors of electronic devices as shown in Fig. 3, where only 2.62% computational time of the high-fidelity model is required with merely a lost of 0.7% accuracy.
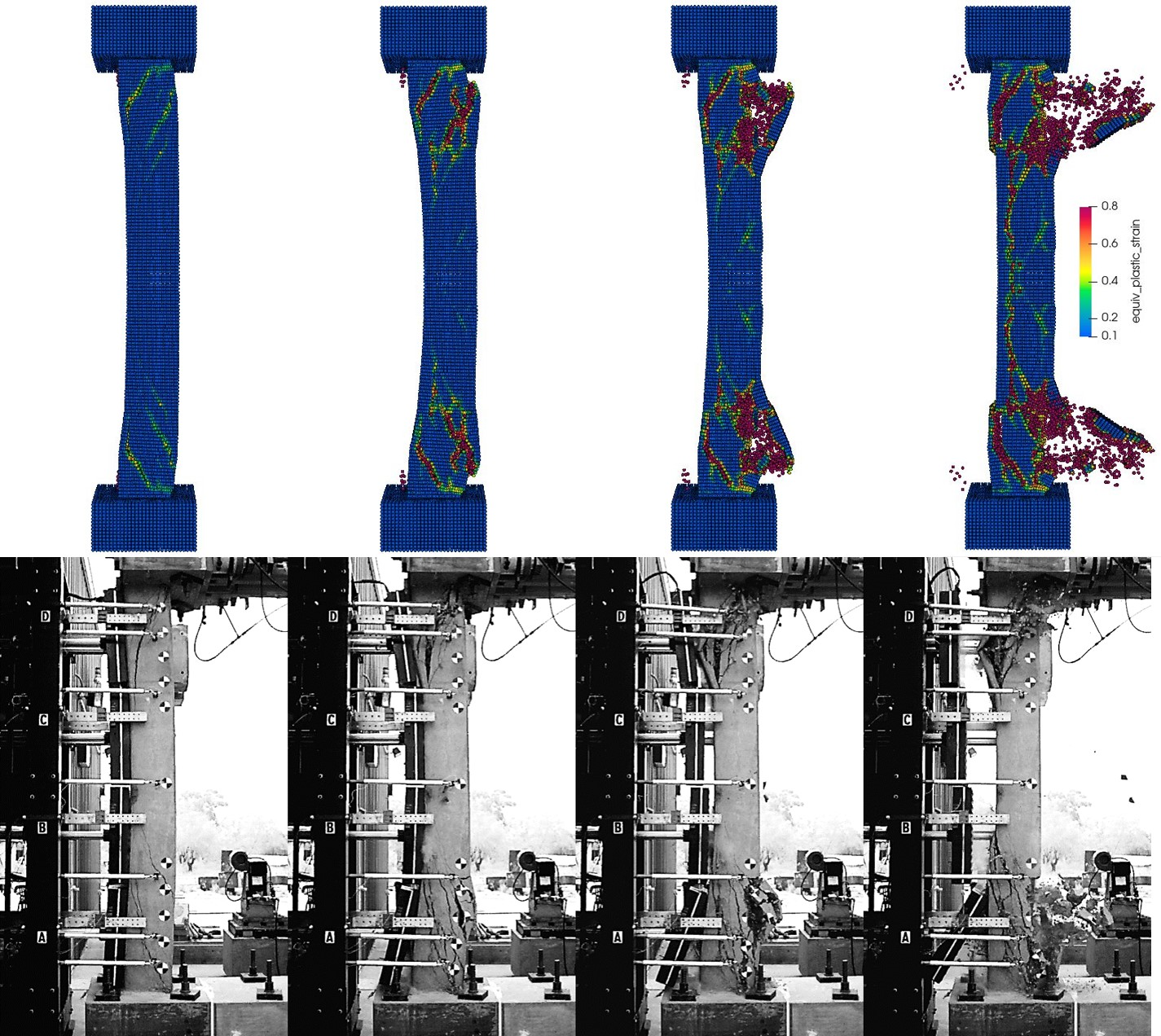
Principal Investigator(s):
Robert J. Asaro
Summary:
The cell wall of S. cerevisae serves to protect the cell from thermal, oxidative and mechanical stresses and it is the target for anti-fungal drugs in pathogenic strains. It also serves as a model for cell wall formation in higher eukaryotes. Little is known about its mechanical properties due to the complex nature of its protein and polysaccharide components, and their interconnections. A multi-scale model describing the cell walls nano-mechanical response to AFM tip indentation and the whole cell’s response to high hydrostatic pressure, nano-indentation and micro-manipulation compression experiments is under development.
Principal Investigator(s):
Qiang Zhu
Summary:
Relying on a unique hybrid locomotion system combining fin flapping and jet propulsion, squids are among the fastest swimmers in the aquatic world. Inspired by this ingenious design, we have developed a novel bio-inspired underwater propulsion system that can achieve high-speed swimming via body deformation and pulsed jetting. Numerical models have been developed to illustrate the underlying physical mechanisms and examine measures to optimize the propulsion performance. Based on these results a prototype has been developed through inter-departmental collaboration. In addition to high speed, this propulsion design also promises high maneuverability (via thrust vectoring) and low environmental footprint, useful features for potential environmental and scientific applications.
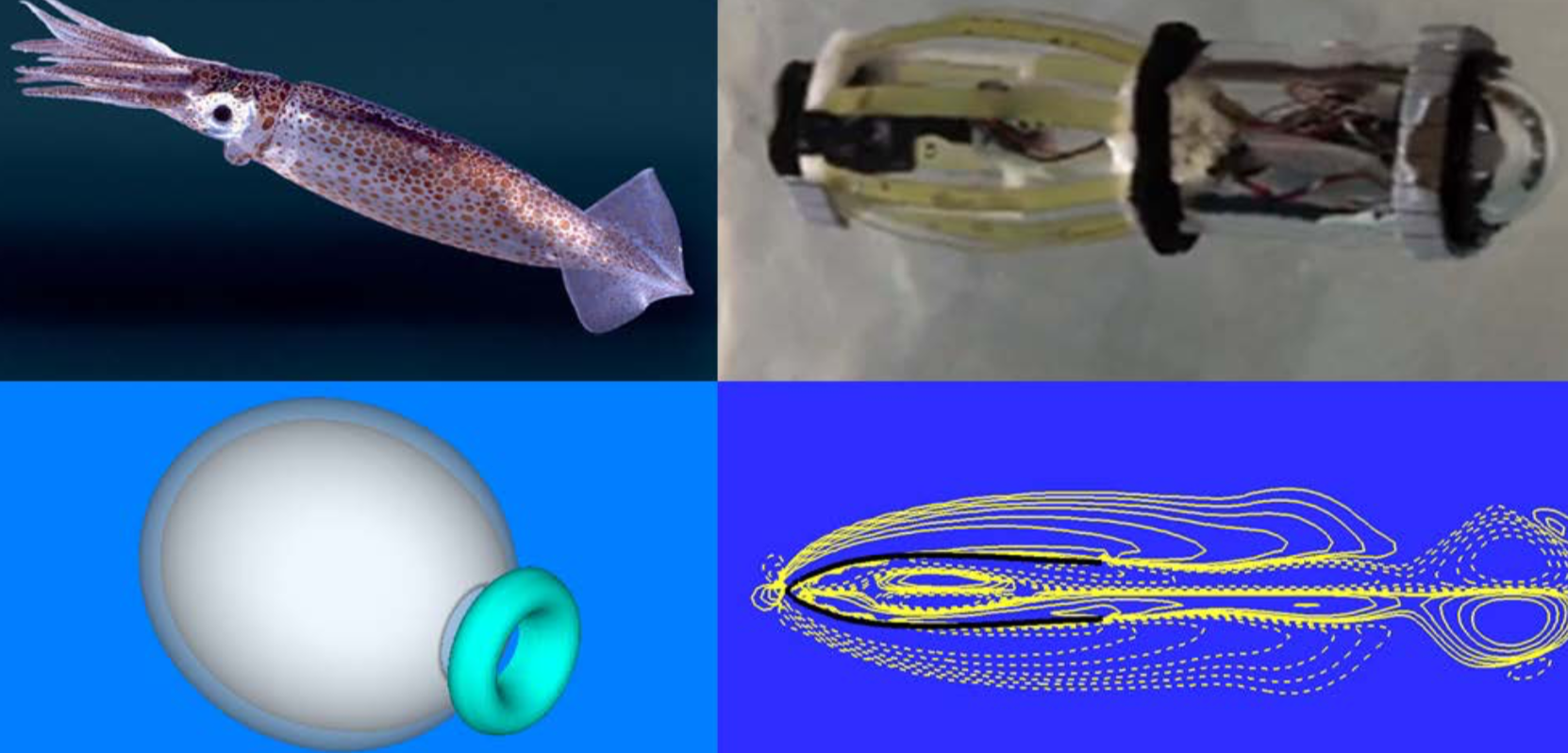
Principal Investigator(s):
Shabnam Semnani
Summary:
Shale is a highly heterogeneous material at multiple scales. A typical shale has a complex microstructure comprised of nanometer-scale pores and minerals mixed with macro-scale fractures and particles of varying size. Computational modeling of this complex and highly heterogeneous rock requires detailed characterization of heterogeneities and microstructure of the material using imaging and visualization techniques. Advances in high-resolution imaging capabilities have made it possible to image heterogeneous materials down to the nano-scale resolution. However, it is generally not feasible to image a large sample of shale at a high resolution over a large field of view (FOV), thus limiting a full characterization of the microstructure of this material. We have developed a statistical framework that uses high-resolution images to enhance low-resolution images obtained over a large FOV. The approach has been demonstrated using X-ray micro-tomography images of organic-rich Woodford shale obtained at different resolutions and FOV.
Multiscale imaging of shale. A single slice of shale with pixel size of 4.14 um, obtained using a low-resolution micro-tomography scan, is shown in (a), and the marked region is enlarged in (b). (c) Illustrates the same region shown in (b) obtained using a micro-tomography scan with a pixel size of 0.517 um. Region 3 marked in (b, c) is enlarged in (d, e), respectively, showing small pyrite particles, framboids, pores/organics, and matrix of low-density minerals and clay, which appear as a blurred range of gray values in the low-resolution image (after Semnani and Borja, 2017)